Laboratory earthquakes demonstrate how grains at fault boundaries cause large quakes.
Caltech engineers have offered substantial experimental support for a kind of seismic propagation presently assumed responsible for the magnitude-9.0 earthquake that destroyed the Japanese shore in 2011.
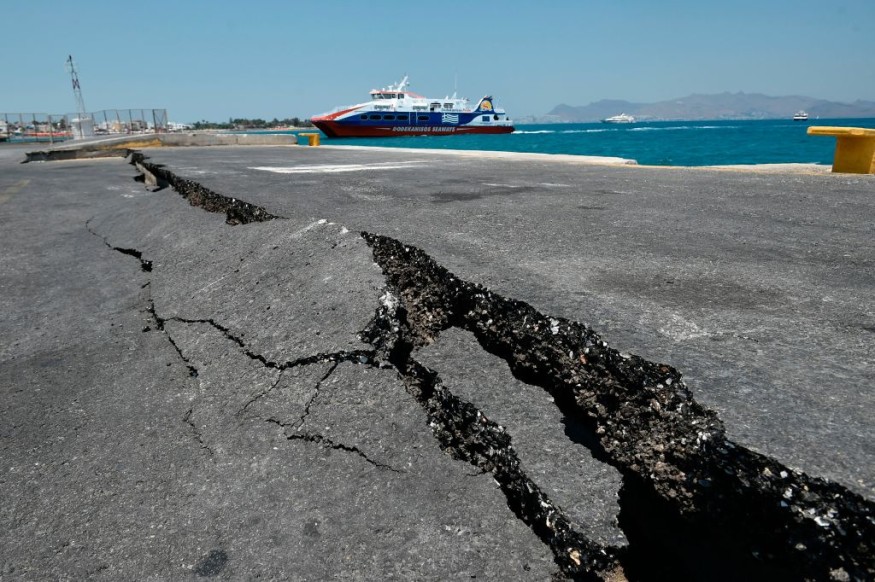
Seismic Simulation
Caltech engineers have offered substantial experimental support for a kind of seismic propagation presently assumed responsible for the magnitude-9.0 earthquake that destroyed the Japanese shore in 2011.
As tectonic plates grind against one another, fine-grained gravel forms along fault lines, which are the borders of tectonic plates. The impact of this gravel on earthquakes has long been a source of debate among scientists.
Caltech researchers illustrate in a new report published in the journal Nature on June 1 that tiny gravel, known as rock gouge, initially stops earthquake propagation but later triggers seismic rebirth, resulting in severe ruptures.
"We were able to look at the earthquake process up close and find essential elements of rupture propagation and friction growth in rock gouge thanks to our new experimental technique," explains Vito Rubino, research scientist and principal author of the Nature study.
"As a result of the activation of co-seismic friction weakening processes, fault sections formerly assumed to operate as barriers against dynamic rupture may host earthquakes," says one of the study's preliminary results.
Intensive Study
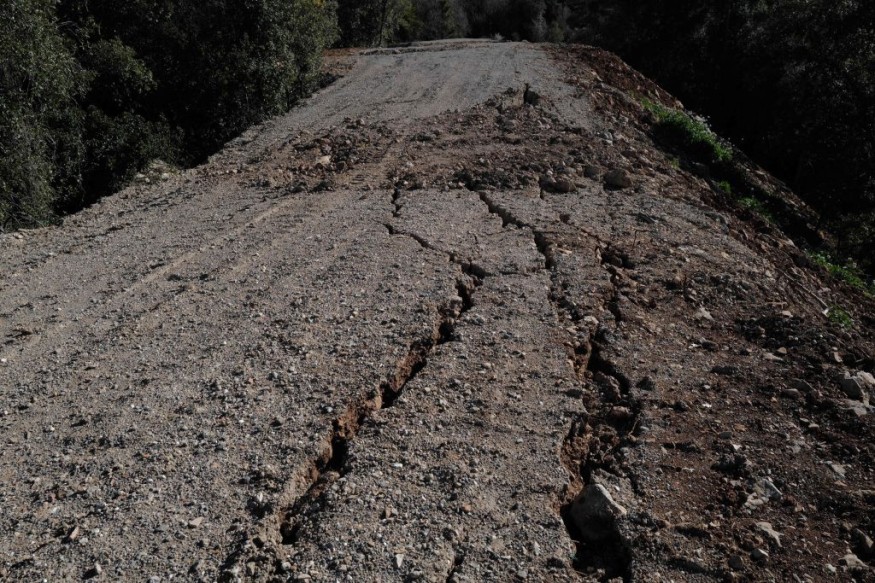
Rubino and his co-authors Nadia Lapusta, Lawrence A. Hanson, Jr., Professor of Mechanical Engineering and Geophysics, and Ares Rosakis, Theodore von Karman Professor of Aeronautics and Mechanical Engineering, show in the paper that so-called "stable" or "creeping" faults are not immune to major ruptures as previously thought.
These faults form when tectonic plates gently glide past one another without causing large earthquakes (for example, the currently creeping section of the San Andreas Fault in central California).
Rock gouge, on the other hand, has a complicated behavior. It works as an initial line of defense against the rupture, collecting energy and preventing it from spreading. The rock gouge interface degrades and drastically decreases friction between the two plates when the plates glide past one other at a high enough velocity, enabling the quake to resurface. Renucleation is the term for this process.
"We know that rock gouge may either strengthen with fault slip and function as a barrier or weaken and encourage earthquake rupture," Lapusta adds, citing a large corpus of past rock-friction tests.
"However, these behaviors are typically thought to be separated in space, with weakening and strengthening occurring on different fault locations. Our experiments show how these behaviors can combine on the same fault locations during the same slip event, over dynamic rupture timescales, resulting in intermittent slip and potentially turning a fault barrier into an earthquake-prone region."
The effect of rock gouge, micrometer-sized granular debris, in seismic activity is investigated in the Nature article. The researchers utilized Caltech's so-called seismological wind tunnel, built by Rosakis and former Caltech Seismological Laboratory director Hiroo Kanamori, to study the influence of rock gouge on earthquake propagation. Emeritus Professor of Geophysics Hazel S. Smits Engineers and scientists may research significant earthquakes on a tiny scale at the facility, which has been operational since 1999.
The team first sliced a translucent meter-sized piece of Homalite in two to create an earthquake simulation. Dynamic rupture nucleation can occur in samples as tiny as tens of centimeters in diameter, whereas examining the same phenomena in rock would need samples of tens of meters in size.
Next, the researchers applied tremendous pressure and shear to the Homalite's two halves, replicating tectonic pressure that builds up along a fault line. Fine-grained quartz powder was inserted between the pieces as a stand-in for fault gouge.
The scientists then placed a short wire fuse between the two pieces, the "epicenter" of the earthquake they were simulating. The high-speed imaging technology was utilized to document the progression of the simulated earthquake one millionth of a second at a time as it developed.
"We could never have imagined its success in discovering such a rich spectrum of physical phenomena relating to frictional earthquake source processes and that such phenomena could be rigorously scaled to explain natural earthquake behavior occurring at massively different length scales around the globe when we were designing the seismological wind tunnel' back in the late 1990s," Rosakis says. "This is a tribute to the discipline of mechanics' immense force."
Needs More Research
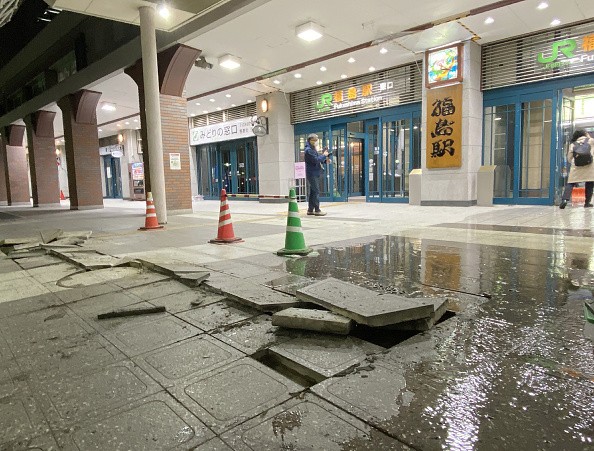
The researchers will then investigate the influence of fluids found naturally in the Earth's crust on the frictional behavior of rock gouges.
Related Article : Experts are Studying the "Biggest Earthquake in Human History"
For similar news, don't forget to follow Nature World News!
© 2024 NatureWorldNews.com All rights reserved. Do not reproduce without permission.